Two-dimensional (2D) materials are crystalline materials that are only one or a few atoms thick. Overall, 2D materials resemble a thin sheet of paper, where the thickness of one axis is on the nanometer length scale. Multiple sheets of 2D materials can be layered together to create a 2D heterostructure. Some classic examples of materials that are 2D materials include graphene, boron nitrides and transition metal dichalcogenides.1
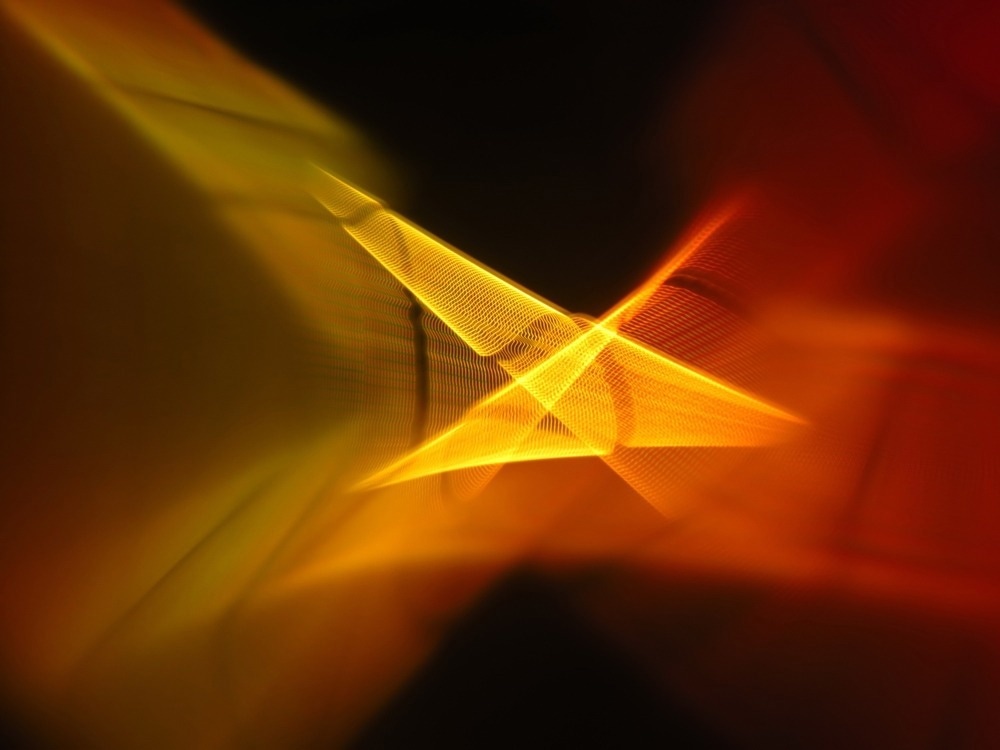
Image Credit: Wirestock Creators/Shutterstock.com
2D heterostructure materials have attracted much scientific and research interest due to their attractive properties for use as sensors, light-emitting diodes and many other applications.1 What makes 2D materials so useful is that many of their properties are size dependent, and the nanoscale nature of one of the dimensions of the material can affect the atomic and elemental properties quite significantly.
For example, in the case of graphene, which was first isolated in 2004, graphene was found to be an excellent electrical conductor.2 Graphene is simply a collection of carbon atoms, which would typically be non-conductive and behave as an electrical insulator at ‘normal’ thicknesses. When one dimension of carbon material is reduced to atomic thickness, the material's electronic structure is changed and delocalized electrons can transport charge through the material.
2D heterostructures are of great interest because they allow not just for property control at the level of the 2D materials used but also additional property tuning with the way the 2D heterostructure is formed. For example, the overall heterostructure behaves as both a bulk material and an interface, making it very different from traditional 3D semiconductor devices, and there is still the possibility of charge transfer through all the layers of the material of interest for ‘band structure engineering’ – where the band gaps are optimized for particular applications.3
While more reliable methods are now available for the creation of 2D materials and their corresponding heterostructures, as the properties of the finished material are highly size-dependent, it is very important to have a characterization method with a sufficient spatial resolution that any defects can be detected as well as the overall topology of the material. Electron microscopy, with its sub-Angstrom resolution, is an ideal way of studying many 2D heterostructured materials and can be used both for structural characterization and understanding the underlying physics behind the properties of the material.4
Electron Microscopy
There are a number of different electron microscopy methods, including transmission electron microscopy (TEM) and scanning electron microscopy (SEM) that can be used to characterize materials on the nanoscale. One aspect that all methods have in common is the use of bright electron beams that are used to bombard the sample. In a TEM experiment, these electrons pass through the sample and are directly detected on a detector placed behind the sample position. In SEM, the scattered electrons are instead detected.
There are some differences in the sample preparation for each method and exactly what information can be collected in the sample images, but both offer superb spatial resolution capable of capturing nanoscale features.
Electron microscopy methods have been applied to the imaging of materials such as hexagonal boron nitride – an excellent electrical insulator and of interest for its optoelectronic properties due to its unusually wide bandgap.5,6 By using scanning tunning electron microscopy (STEM) imaging approaches, the team could identify the junctions between the graphene and the boron nitride heterostructures and see how certain defects could induce strain in the structure identify many of the angles involved in the lattice structure of the material.5
One of the challenges with using TEM to study 2D materials has been the knock-on damage caused by the intense electron beams.7 Refinement of the experimental conditions, such as reduction of the electron acceleration voltages, can help reduce this and help with obtaining aberration-free images.
Electron microscopy methods are also being combined with other electrical approaches so the microstructural changes in the materials can be analyzed under something close to ‘operating’ conditions, as would be found in optoelectronic devices.7
Optoelectronics
One of the major promising application areas for heterostructures of 2D materials is the field of optoelectronics. Optoelectronics devices are electronic devices that can be used to control light and include everything from the devices involved in fiber optic communications, a number of sensor types, solar cells and lasers.
The tunability of the optical properties of 2D materials and their heterostructures is one aspect of what makes 2D materials so exciting for optoelectronics applications, but also that many materials are not just strong absorbers but have good charge transport properties as well.
2D materials such as black phosphorous are making it possible to design optoelectronic devices that operate in regions of the electromagnetic spectrum that are challenging to cover with traditional materials.8 Transition metal dichalcogenides, like MoS2 and WSe2, have very high carrier mobilities and their band gap is tunable depending on the thickness of material. The size dependence of these properties, therefore, makes it incredibly important to also develop electron microscopy methods for the characterization of these thicknesses so full control over the design and fabrication processes can be realized.
References and Further Reading
Wang, H., Liu, F., Fu, W., Fang, Z., Zhou, W., & Liu, Z. (2014). Two-dimensional heterostructures: fabrication, characterization, and application. Nanoscale, 6, pp. 12250–12272. https://doi.org/10.1039/c4nr03435j
Novoselov, K. S., Geim, A. K., Morozov, S. V., Jiang, D. E., Zhang, Y., Dubonos, S. V., ... & Firsov, A. A. (2004). Electric field effect in atomically thin carbon films. Science, 306(5696), pp. 666-669. https://doi.org/10.1126/science.1102896
Novoselov, K. S., Mischenko, A., Carbalho, A., & Netro, A. H. C. (2016). 2D materials and van der Waals heterostructures. Science, 353, p. 6298. https://doi.org/10.1126/science.aac9439
Smith, D. J. (2008). Ultimate resolution in the electron microscope? Materials Today, 11, pp. 30–38. https://doi.org/10.1016/S1369-7021(09)70005-7
Roy, S., Zhang, X., Puthirath, A. B., Meiyazhagan, A., Bhattacharyya, S., Rahman, M. M., Babu, G., Susarla, S., Saju, S. K., Tran, M. K., Sassi, L. M., Saadi, M. A. S. R., Lai, J., Sahin, O., Sajadi, S. M., Dharmarajan, B., Salpekar, D., Chakingal, N., Baburaj, A., … Ajayan, P. M. (2021). Structure , Properties and Applications of Two-Dimensional Hexagonal Boron Nitride. Advanced Materials, 33, p. 2101589. https://doi.org/10.1002/adma.202101589
Zan, R., Ramasse, Q. M., Jalil, R., Tu, J.-S., Bangert, U., & Novoselov, K. S. (2017). Imaging Two Dimensional Materials and their Heterostructures Imaging Two Dimensional Materials and their. Electron Microscopy and Analysis Group Conference, 902, p. 012028.
Chang, Y., Han, H. N., & Kim, M. (2019). Analyzing the microstructure and related properties of 2D materials by transmission electron microscopy. Applied Microscopy, 49, p. 10. https://doi.org/10.1186/s42649-019-0013-5
An, J., Zhao, X., Zhang, Y., Liu, M., Yuan, J., Sun, X., Zhang, Z., Wang, B., Li, S., & Li, D. (2022). Perspectives of 2D Materials for Optoelectronic Integration. Advanced Functional Materials, 32, p. 2110119. https://doi.org/10.1002/adfm.202110119
Disclaimer: The views expressed here are those of the author expressed in their private capacity and do not necessarily represent the views of AZoM.com Limited T/A AZoNetwork the owner and operator of this website. This disclaimer forms part of the Terms and conditions of use of this website.