The excellent electrical properties of semi-metallic graphene have made it an irreplaceable material in many applications. Opening the band gap in graphene without compromising on its other established properties would be a huge revolution to the semiconductor industry. Reports indicate that graphene semiconductors could offer a replacement for the silicon industry.
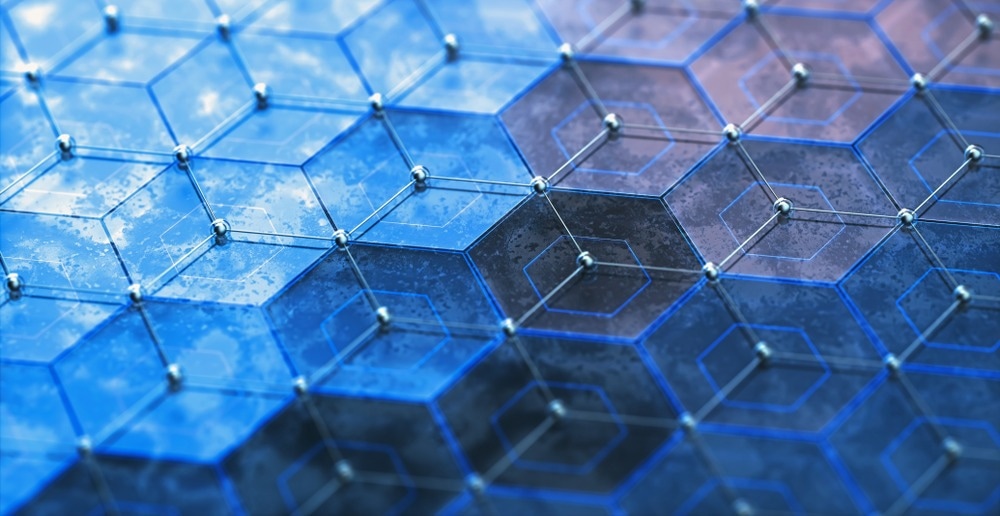
Image Credit: ktsdesign/Shutterstock.com
Exfoliation of graphite into single layers called graphene was a revolutionary discovery, opening many doorways to material exploration and applications. Graphene contains a carbon chain linked in sp2 hybridization, making it a material with high electrical conductivity.
The charges, electrons, or holes achieve ballistic transport pathways in the direction of the plane of the graphene layer at room temperature, recording the highest charge mobility ever recorded in any material.
Exceptional properties of graphene, such as high mechanical flexibility, strength, and physical and chemical stability, make it the optimum material for vast applications. Graphene is a zero-bandgap material semiconductor, also called a semimetal. This intrinsic nature of graphene impedes its application in many electronics.
Hence, the scientific community has investigated the possibilities of transforming graphene into a semiconductor that could open up various applications and enhance the performance of many existing devices. Semiconducting graphene has the potential to replace silicon in the future, as the downscaling of silicon has reached its limit.1
Understanding Graphene as a Semiconductor
The delocalized bonding (p) and anti-bonding (p*) bands of graphene degenerate at the Dirac point of the Brillouin zone. The two bands (valence and conduction band) have linear dispersion and are considered two cones touching at the Dirac point, forming a zero-band structure.
This zero-band structure results from the identical environment of the two carbon atoms in a single unit cell of the hexagonal crystal lattice. Hence, to open up the band structure, researchers came up with the idea of destroying the symmetry of the carbon structure by structural or chemical modifications.
Many strategies are employed to make graphene semiconductors. For example, nitrogen replaces carbon to disturb the symmetry in the hexagonal lattice, creating an optical bandgap.
Another example is the patterning of the graphene sheets into one-dimensional nanoribbons, two-dimensional nano-mesh, or zero-dimensional quantum dots. The edges the structure created to form nanoribbon and quantum dots break the symmetry in the lattice and leads to the formation of band gap.
Changing the stacking order of the graphene layers to Bernel stacking and applying mechanical deformation/strain on the graphene lead to symmetry breaking in the graphene lattice, resulting in the bandgap opening.1,2
Properties of Graphene Semiconductors
Due to the quantum confinement effect, the energy band structure of semiconducting nanomaterials shows size-dependent transformations. Due to the one-dimensional confinement, the electronic band structure changes inversely with the nanoribbon width in a semiconducting graphene nanoribbon.
E-beam lithography was mostly employed to control patterning on graphene sheets to create graphene nanoribbons, mesh, and quantum dots. Various feature sizes of this patterned graphene were reported in a nanoscale regimen, with bandgap values reporting from ~ 0.05 eV (in the case of nanoribbons) to ~ 0.5 eV (in the case of quantum dots).
A recent Nature publication, reported by a team from Georgia Institute of Technology, USA, led by Walt A de Heer, demonstrated the growth of semiconducting epigraphene (SEG) on single crystal silicon carbide substrates. They reported the material with a bandgap of 0.6 eV and an electron transport mobility of 5000 cm2. V-1.s-1 is much larger than the mobility reported by silicon and other two-dimensional semiconductors.
This semiconducting graphene is chemically, mechanically, and thermally stable. They reported a unique fabrication technique demonstrating a well-ordered layer over a flat terrace of silicon carbide substrate.3,4
Applications of Graphene Semiconductors
Semiconducting graphene with a tunable bandgap can be applied in many applications in electronic devices. Along with the excellent electrical properties, the graphene semiconductor has implications in photonics, opto-electronics, sensors, fuel cells and solar energy harvesting, thermos-electric devices, supercapacitors, and lithium-ion batteries.
Conventional semiconductors mostly lag in the efficient transport of the charge carriers. However, graphene semiconductors, with excellent charge mobility, could enhance the response time of several devices, such as field effect transistors (FETs).2
Advantages and Challenges
Such explorations in graphene will promote research in other two-dimensional materials and its heterostructures. This can open up doors for fundamental research and will lead to performance enhancement in several devices.
The precise control over the size, shape and edges of the graphene-based nanostructures, such as nano-ribbons, nano-mesh and quantum dots, is still challenging for the research community.
Along with the opening of the bandgap, maintaining the electrical properties of the material is crucial, and synthesis of defect-free materials could help to achieve high carrier mobility of the charge carriers.
Significant development of semiconductor graphene is vital and expected in the near future. This would lead to the replacement of less efficient silicon from the semiconducting industry.
References and Further Reading
- Lu, G., Yu, K., Wen, Z. and Chen, J., 2013. Semiconducting graphene: converting graphene from semimetal to semiconductor. Nanoscale, 5(4), pp.1353-1368.
- Maharubin, S., Zhang, X., Zhu, F., Zhang, H.C., Zhang, G. and Zhang, Y., 2016. Synthesis and applications of semiconducting graphene. Journal of Nanomaterials, 2016.
- Zhao, J., Ji, P., Li, Y., Li, R., Zhang, K., Tian, H., Yu, K., Bian, B., Hao, L., Xiao, X. and Griffin, W., 2024. Ultrahigh-mobility semiconducting epitaxial graphene on silicon carbide. Nature, 625(7993), pp.60-65.
- Nevius, M.S., Conrad, M., Wang, F., Celis, A., Nair, M.N., Taleb-Ibrahimi, A., Tejeda, A. and Conrad, E.H., 2015. Semiconducting graphene from highly ordered substrate interactions. Physical review letters, 115(13), p.136802.
Disclaimer: The views expressed here are those of the author expressed in their private capacity and do not necessarily represent the views of AZoM.com Limited T/A AZoNetwork the owner and operator of this website. This disclaimer forms part of the Terms and conditions of use of this website.