Researchers from The University of Queensland, Australia, have engineered a new material that is a promising candidate to be implemented into the next generation of electronics with advanced features. This new carbon-based material is expected to expand the capabilities of nanoscale electronics and contribute to the growth of the nanoelectronics market in the coming years.
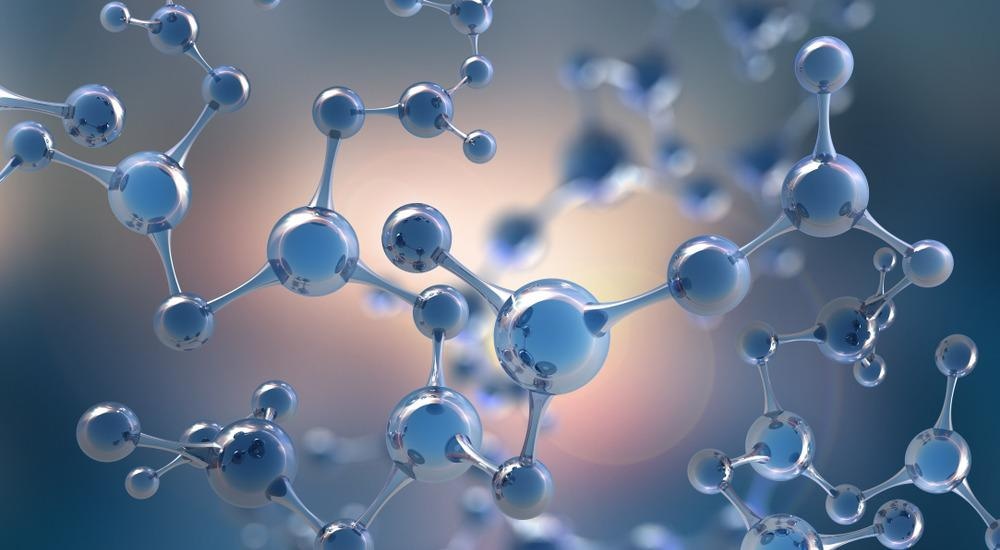
Image Credit: Yurchanka Siarhei/Shutterstock.com
Carbon Nanomaterials for Electronics Applications
Graphene and other carbon nanomaterials are of interest both to academia and industry for their applications in electronic devices. Carbon-based materials have many properties that are desired in the field, such as high mechanical strength as well as good thermal and electrical conductivity.
Significant progress has been made in the past few decades, using graphene nanoribbons and carbon nanotubes to create new electronics that are used in various aspects of our lives, from communication to computing. These advances have often been a result of the reduction in the size of the electronic devices which has led to faster and more efficient circuitry.
Challenges for Carbon-Based Nanomaterials
Although these technological advances have been crucial for the fast development of the industry, there are still many technical challenges that remain. These challenges still restrict the widespread application of carbon-based electronics.
One such challenge is the generation and modulation of bandgaps in carbon-based materials. For example, in the fabrication of field-effect transistors (FET), it is very important for the material to possess an electronic bandgap. When producing FET, semiconducting carbon nanotubes are often used because they have a medium-sized bandgap.
Graphene for Electronics Applications
Ever since its discovery in 2004, graphene has been of interest to material scientists and engineers for its numerous applications in fields ranging from healthcare to photonics and electronics. With its high carrier mobility at low temperatures, graphene is one of the most promising materials for high-speed transistors.
A great limitation to the commercial production of graphene-based electronics is that graphene does not have a bandgap. Hence, it cannot be used for FETs unless it is patterned into nanoribbons with zigzag edges, to allow for tunable bandgap. However, the fabrication of nanoribbons is still facing many challenges related to both their production and functionality.
Carbon-Nitride Crystalline Structures
The new material C3N bilayers, engineered by the Australian Institute for Bioengineering and Nanotechnology (AIBN) and the School of Chemistry and Molecular Biosciences (SCMB) from The University of Queensland, offers a solution to many of these challenges. The team's research was published in the journal Nature Electronics.
The concept of this new material was originally created by Dr Qinghong Yuan, a researcher at AIBN. It was developed by researchers at SCMB and AIBN, and the theoretical calculations were performed by Wenya Wei, a visiting PhD student.
C3N bilayers are obtained by substituting some of the carbon atoms from two layers of the honeycomb lattice with nitrogen atoms.
The resulting material has a bandgap and transforms into an n-type semiconductor. Crystalline C3N has been theoretically predicted to be a stable semiconductor 2D material. However, most synthesized carbon-nitride films are not suitable for nanoelectronics applications since it is very difficult to obtain a highly regular two-dimensional carbon-nitride crystalline structure.
Fabrication of 2D Semiconductors
The fabrication of the 2D semiconductor can be performed by engineering its optical and transport properties using Van der Waals coupling. The interlayer interactions can be controlled either by applying an external field or by designing interlayer stacking configurations.
The bandgap of most 2D carbon-based semiconductors decreases with increasing thickness. Although carbon-based materials traditionally show limited bandgap modulation, several 2D materials, including C3N bilayers, can exhibit a widely tunable bandgap.
The twist angle between neighboring layers can be changed if atomic force microscope tip manipulation is used. Changing the alignment of the layers can result in the ability to adjust the electricity flow in some electronic devices, which is not possible when using just graphene.
Further Developments
The new structures developed by the researchers from AIBN and SCMB will enable the production of new types of electronic components which could be used in various existing technologies. For example, they can be applied in a wide range of products, from smartwatches to refrigerators, offering better performance. It can also be applied in flexible electronics as well as computer memory.
The researchers working on this project are very excited to have engineered a material that matches their theoretical predictions. Although the development of this carbon-based semiconductor is very promising, Professor Bernhardt shares that there is still a lot of work to be done to increase scalability so that the material is widely available to industries at a reduced cost.
References and Further Reading
De Wit, E. (2021) Smarter electronics a step closer with nanotech advance. [Online] The University of Queensland. Available at: https://www.uq.edu.au/news/article/2021/06/smarter-electronics-step-closer-nanotech-advance (Accessed on 21 July 2021).
Wei, W., et al. (2021) Bandgap engineering of two-dimensional C3N bilayers. Nature electronics. https://doi.org/10.1038/s41928-021-00602-z
Avouris, P., Chen, Z. and Perebeinos, V. (2007) Carbon-based electronics. Nature nanotechnology. https://doi.org/10.1038/nnano.2007.300
Li, Y. Y., Chen, M. X., Weinert, M. and Li, L. (2014) Direct experimental determination of onset of electron–electron interactions in gap opening of zigzag graphene nanoribbons. Nature communications. https://doi.org/10.1038/ncomms5311
Disclaimer: The views expressed here are those of the author expressed in their private capacity and do not necessarily represent the views of AZoM.com Limited T/A AZoNetwork the owner and operator of this website. This disclaimer forms part of the Terms and conditions of use of this website.