Graphene is a single-atom-thick carbon sheet discovered in 2004. The crucial question during graphene synthesis is, "How do I know that the material I'm making is actually single-atom thick?" Because without an understanding of what you're creating, it's impossible to put it to good use, which is where spectroscopy comes in.
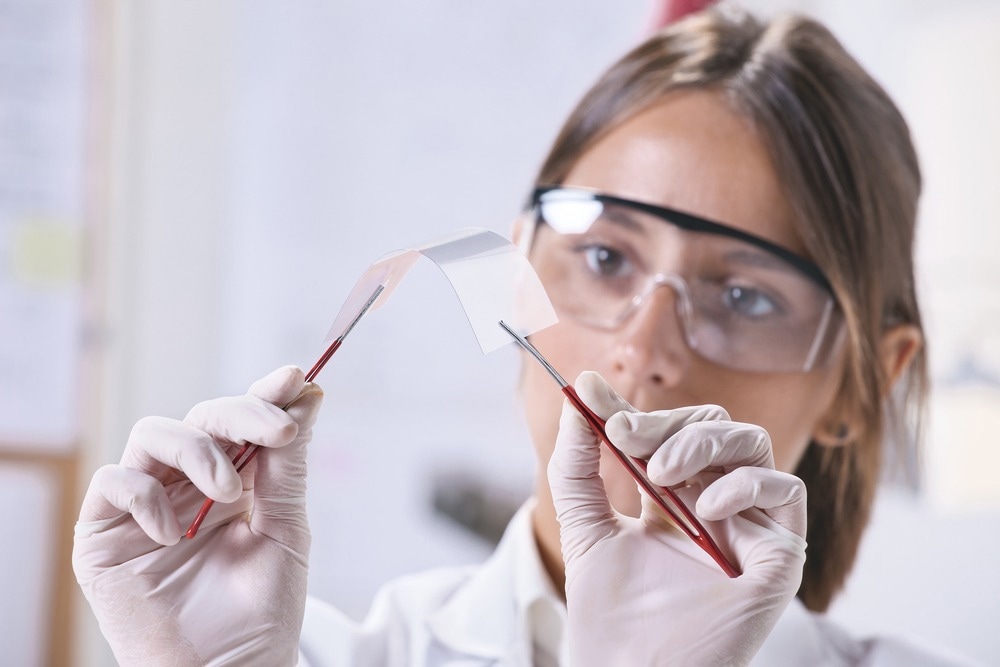
Image Credit: BONNINSTUDIO/Shutterstock.com
This article aims to provide insight into the most common and impactful spectroscopy methods for characterizing graphene– it should be pointed out, however, that this list is not entirely complete.
Spectroscopy is a technique for studying the interaction of light and matter to determine the structure and properties of nanomaterials. Graphene loves to interact with electromagnetic radiations. The amount of radiation absorbed or emitted by graphene can be used to determine its components, optical and electrical properties, structural composition, and crystal structure of graphene. Given the variety of approaches available in the field, we will concentrate on the broader spectroscopic techniques that aid in graphene characterization.
X-Ray Diffraction Spectroscopy (XRD)
XRD is an important tool for analyzing graphene's crystallite size, interplanar spacing, and crystalline structure. A high-intensity peak at 2θ = 26.6 corresponds to pristine graphitic structure typically observed in XRD. This peak confirmed graphite's well-organized layered structure, with an interlayer spacing of 0.34 nm along (002) orientations. Another critical function of XRD is to ensure that GO is reduced to graphene. As graphite oxidizes to GO, the two peak shifts to the left to 10.02°, increasing the interplanar distance to 0.9 nm.
Following the successful reduction, oxygen-containing functional groups are eliminated, and the peak at 10.02° vanishes completely. In contrast, a broader peak at 24–26° corresponding to the (002) plane of reduced GO appears, indicating the restoration of graphene's -conjugated structure due to reduction. Furthermore, the interplanar spacing of rGO is reduced to 0.36 nm, indicating that oxygen-containing functional groups are removed.
X-Ray Photoelectron Spectroscopy (XPS)
XPS is a powerful surface-sensitive spectroscopic technique that can be used to determine the elemental composition and chemical bonding of graphene derivatives. The sp2 carbon fraction, which is influenced by the presence of functional groups, is the most critical parameter for determining the degree of oxidation or reduction.
There are two prominent peaks at the binding energies of 284 and 530 eV, commonly known as C1s and O1s peaks, in the XPS spectra of GO and rGO, respectively. The C1s peak is further deconvoluted for functional groups containing oxygen at 284.5 eV (C-C), 286.4 eV (C-O), 287.8 eV (C=O), and 289.0 eV (COOH). The percentage of functional groups present is determined by the area covered by the peaks. There are numerous examples of using XPS to investigate the surface of reduced graphene oxide and graphene oxide, the composition of graphene quantum dots, functionalized graphene nanosheets, and so on.
Ultraviolet Photoelectron Spectroscopy (UPS)
In ultraviolet photoelectron spectroscopy (UPS), UV photons are used to eject an electron from the graphene, which can only eject an electron from the valence band because of their limited depth of penetration, which is only a quarter that of X-rays.
UPS is frequently used to confirm the results of band structure calculations by determining the shift in work function, valence band structure, and electronic structure of graphene, particularly the value of the Fermi level. For example, in one of the case studies, researchers reported that N-type doping of the nitrogen atoms in graphene reduces the work function by 0.3 eV compared to pristine graphene.
Ultraviolet (UV)-Visible Spectroscopy
UV–Visible spectroscopy is used to detect the conjugation network and GO and rGO absorption. UV-Vis is frequently used to detect GO in suspension. The absorption peak in the UV spectra of GO is at 225-233 nm, which is shifted to 270 nm in rGO. The absorption peak at 230 nm is due to the π-π* transition of an aromatic C–C ring. In contrast, the UV spectra of rGO show a red shift at 270 nm. This absorption peak is attributed to the n-π* transition of C–O bonds.
Fourier-Transform Infrared Spectroscopy (FTIR)
FTIR is used to determine the presence or absence of functional groups on GO and rGO sheets by measuring the absorbance of infrared light energy at different wavelengths. There are no significant peaks in the FTIR spectrum of pristine graphene. However, characteristic peaks for CO bonds can be seen in the GO spectra at approximately 1230–1215 cm−1, 1415 cm−1, 1160 cm−1 (from carbonyl or carboxyl), 1120–1110 cm−1 (from epoxy), and 1080–1040 cm−1 (from alkoxy or epoxy). In addition, the peak corresponding to the hydroxyl (H‒H) groups can be seen in the 4000–3000 cm−1 range.
Raman Spectroscopy
Raman spectroscopy is a widely used and highly sensitive inelastic scattering of light technique for evaluating graphene's electrical, phonon, and optical properties. Graphene's Raman spectra contain three notable peaks of interest: the 2D peak, the G-peak, and the D-peak.
At approximately 2700 cm-1, the 2D-peak (also known as the G' peak) appears. When the graphene thickness increases from single layer graphene (SLG) to multilayer graphene (MLG), the 2D peak broadens and blue shifts (MLG). The G-peak is roughly at 1585 cm-1, and its intensity increases as the number of layers increases.
The disorder band, which occurs between 1270 and 1450 cm-1, is commonly referred to as the D peak. The D peak can also be used to identify defects in graphene-based materials such as graphene oxide, disordered graphene, and nanographene. The intensity ratio (I2D/IG) and the FWHM of the 2D peak can be used to calculate the number of graphene layers. With high-quality SLG, for example, the I2D/IG ratio will be 2 and the FWHM of the 2D band will be close to 30 cm-1.
In general, Raman spectroscopy can be used to determine structural information such as the number of graphene layers, the impact of defects or disorders, residual stress, and doping level. External perturbations such as doping, defects, stress, strain, temperature, and magnetic fields cause dramatic changes in graphene's electrical and lattice vibration properties, which can be measured using Raman spectroscopy.
References and Further Reading
Bo, Z., et al. (2014) Green preparation of reduced graphene oxide for sensing and energy storage applications. Scientific reports, 4(1), 1-8.
Wu, J., et al. (2018) Raman spectroscopy of graphene-based materials and its applications in related devices. Chemical Society Reviews, 47(5), pp.1822-1873.
Yang, H., et al. (2011). Influences of graphene oxide support on the electrochemical performances of graphene oxide-MnO2 nanocomposites. Nanoscale Research Letters, 6(1), 1-8.
Boutchich, M., et al. (2014). Atmospheric pressure route to epitaxial nitrogen-doped trilayer graphene on 4H-SiC (0001) substrate. Applied Physics Letters, 105(23), 233111.
Lai, Q., et al. (2012). Ultraviolet-visible spectroscopy of graphene oxides. Aip Advances, 2(3), 032146.
Ţucureanu, V., et al. (2016). FTIR spectroscopy for carbon family study. Critical reviews in analytical chemistry, 46(6), 502-520.
Disclaimer: The views expressed here are those of the author expressed in their private capacity and do not necessarily represent the views of AZoM.com Limited T/A AZoNetwork the owner and operator of this website. This disclaimer forms part of the Terms and conditions of use of this website.