Graphene is widely popular among two-dimensional (2D) materials due to its exceptional physical and electronic properties. Its bilayer form is synthesized by stacking two graphene monolayers; twisting these layers by a small angle results in a twisted bilayer graphene (tBLG) superlattice.1
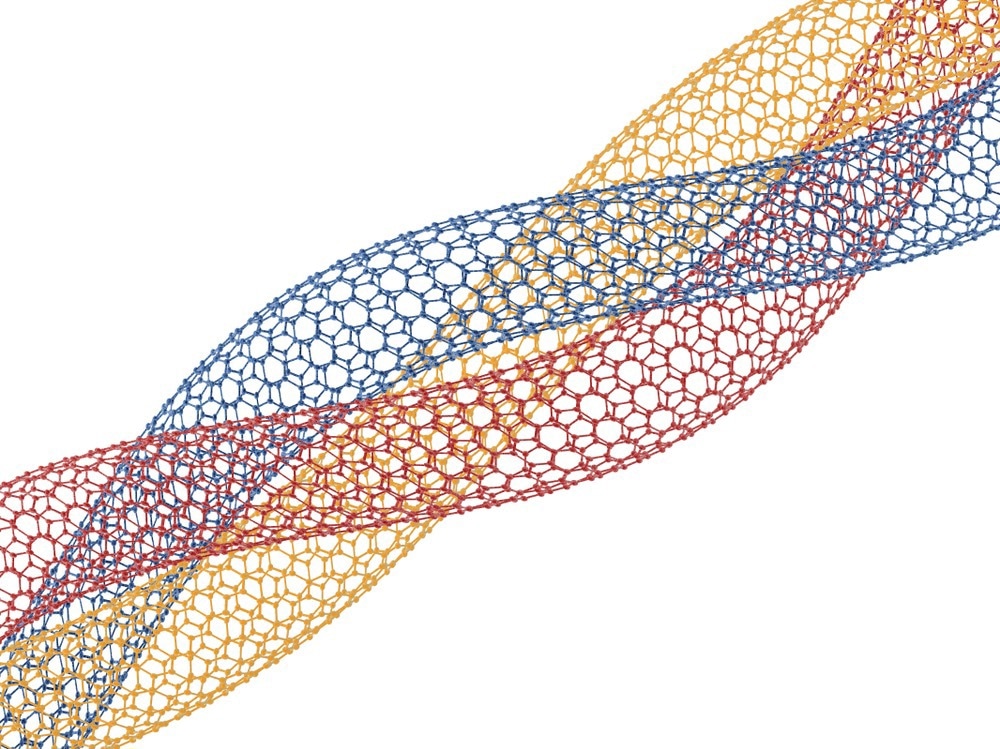
Image Credit: Angel Soler Gollonet/Shutterstock.com
The observation of the moiré pattern due to relative orientation between these stacked layers has prompted rapid advances in graphene research.2 The disorders and interlayer interactions in tBLG enhance its optical and electrical properties, including superconductivity.1 This article explores recent breakthroughs in tBLG and its potential applications in material science and beyond.
Fundamentals of tBLG
The mechanical, optical, and electronic properties of multilayer graphene structures can be tailored by altering the stacking order, interlayer spacing, and relative twisting angle (θ).1 Similarly, tBLG is fabricated by stacking two single-layer graphene sheets, synthesized by chemical vapor deposition (CVD), at a specific twisting angle.1
The unusual stacking imparts various angle-dependent properties to tBLG.2
Moiré patterns are generated by graphene-graphene interactions resulting from the relative layer orientations. These highly periodic patterns are responsible for the extraordinary optical and electronic properties of tBLG. Additionally, tBLG exhibits twisting angle-dependent Dirac spectra (similar to chirality dependence in carbon nanotubes), Fermi velocity, magnetoresistance oscillations, and quantum Hall effect.1
The optical and electrical properties of tBLG devices are governed by the twisting angle between the two graphene layers. At a particular "magic angle," tBLG demonstrates extraordinary physical characteristics such as superconductivity, correlated insulation, and density waves.2 Moreover, tBLG systems display high conductivity variation, revealing a transition from semiconducting to metallic behavior.1
Other unique characteristics of tBLG include the low-lying flat bands around the magic angles. Additionally, tBLG’s Fermi energy is lower than 10 meV around the magic angle (θ = 1.05 degrees); however, the superconducting critical temperature of about 1 Kelvin is relatively high. Such unconventional behavior of tBLG is intriguing for the research community.1
Breakthrough Discoveries
Innovative methods are being explored to fabricate tBLG with small twist angles. For example, hexagonal boron nitride is utilized to obtain graphene layers with rotationally aligned crystal axes. tBLG is also prepared by cutting, rotating, and stacking a graphene layer through femtosecond laser micromachining and precise transfer.
Other methods for preparing tBLG films include controlled hydrophilic and hydrophobic boundary folding of single-layer graphene and vertical stacking.1
Easy twisting and stacking of two graphene layers can result in a uniform and ordered moiré superlattice capable of exhibiting unusual superconductivity and correlations in tBLG. However, the twist angle becomes rigid after interlayer stacking. Alternatively, mechanical elastic strain can help control the electronic structure of tBLG by regulating the lattice spacing and symmetry.
A recent article in Small reviewed various innovations in straining tBLG by in-plane and out-of-plane modes. It included the characterizations and calculations performed to quantitatively tune the strain-engineered electronic structures.3
Another recent study in 2D Materials demonstrated a topological superconducting state in tBLG depending only on the moiré minibands instead of the twist angle tuning. The method involved subjecting tBLG to induced Rashba spin-orbit coupling, s-wave superconductivity, and exchange field and is valid for 1.3 to 3 degrees twist angles. This approach could be feasible for developing a tBLG-based quantum computer.4
Technological Applications and Potential
Tunable interlayer linking and band architecture of tBLG hold immense potential for advancing tBLG-based devices, advanced materials, and different quantum phenomena.1,2 Mechanically stacked t-BLG can also help differentiate its superconducting mechanism from conventional superconductors, potentially enabling the development of materials exhibiting superconductivity at near-ambient temperatures.2
Novel straining modes like shearing and bending extend elastic strain engineering beyond the traditional tension mode, opening avenues for developing flexible, strain-tailored tBLG electronic devices. For example, applying deep strain on tiny twisted tBLG shifts its hexagonal soliton structure to a one-dimensional network, which modulates its electrical properties.2
A recent study in Nature Communications demonstrated silicon photonics (SiPh) waveguide-integrated tBLG photodetectors. The developed detector exhibited a responsivity of 0.65 A/W for 1550 nm telecom wavelength, enabling a 3-dB bandwidth and 50 Gbit/s data stream rate. The exceptional photodetector performance was credited to the high optical absorption of tBLG with a 4.1-degree twist angle.
Overall, this study underscores the potential of large-area tBLG for heterogeneous integration with SiPh.5
How Have Women Shaped Graphene Research in the Past Year?
Challenges
Despite several research advances and potential applications, significant challenges remain in achieving controlled twisting of two graphene layers to fabricate and characterize tBLG.1 Additionally, precise stacking of bilayer graphene at the first magic angle of 1.1 degrees to observe superconductivity is tedious due to the intrinsic disruptions caused by strain and angular disorder.3
Modifications in the twist angle significantly alter the spatial wavefunction distribution. While this allows engineering bandgaps in tBLG for electronic applications, the localized wavefunction at certain twist angles leads to a sudden reduction in carrier mobility. This can negatively influence the tBLG-based device performance. Hence, careful considerations are required to obtain preferred electronic characteristics and carrier mobility for ideal device functioning.2
Scanning tunneling microscopy (STM) has been widely employed to comprehend the atomic and electronic structures of tBLG. However, higher-resolution instruments and techniques are required for further advancements and applications in this field. Techniques like angle-resolved photoemission spectroscopy are being proposed to delve deeper into the unique characteristics of tBLG and the underlying physics.2
The properties of twisted 2D materials like graphene are tailorable using strain engineering. However, achieving precise and uniform strain control over large areas poses significant technical hurdles.
The ultrathin nature of tBLG leads to localized stretching or compression due to substrate deformation or thermal stresses during fabrication. Despite the positive impact of these localized strains on the physical properties of the material, their non-uniformity hinders practical device applications of the material.3
Future Prospects
The constant improvements in the synthesis, characterization, and electronic structure determination techniques are anticipated to accelerate the tBLG-related advances and applications. This, in turn, may lead to the advancement of "twistronics," the fusion of "twist" and "electronics," which exploits the electronic properties of layered materials like graphene changing with the twist angle.2
The strain engineering of twisted 2D materials like tBLG can further expand "twistronics" and "straintronics" into the realm of "strain-twistronics." Consequently, novel ferroelectric and optoelectronic devices with tunable characteristics and regulated performance may become a reality in the future.2
A highly promising frontier for tBLG is superconductivity. The behavior of magic-angle tBLG is similar to that observed in high-temperature superconductors. A comprehensive understanding of the intrinsic mechanisms of these 2D materials can open up avenues for developing high-temperature superconducting materials, novel gate-defined Josephson junctions, and superconducting quantum interference devices (SQUIDs).2
More from AZoNano: Can Semiconductor Chips be Recycled?
References and Further Reading
- Nimbalkar, A., Kim, H. (2020). Opportunities and Challenges in Twisted Bilayer Graphene: A Review. Nano-Micro Letters. doi.org/10.1007/s40820-020-00464-8
- Dindorkar, SS., Kurade, AS., Shaikh, AH. (2023). Magical moiré patterns in twisted bilayer graphene: A review on recent advances in graphene twistronics. Chemical Physics Impact. doi.org/10.1016/j.chphi.2023.100325
- Hou, Y., Zhou, J., Xue, M., Yu, M., Han, Y., Zhang, Z., Lu, Y. (2024). Strain Engineering of Twisted Bilayer Graphene: The Rise of Strain‐Twistronics. Small. doi.org/10.1002/smll.202311185
- Khosravian, M., Bascones, E., Lado, J. (2024). Moiré-enabled topological superconductivity in twisted bilayer graphene. 2D Materials. doi.org/10.1088/2053-1583/ad3b0c
- Wu, Q., et al. (2024). Waveguide-integrated twisted bilayer graphene photodetectors. Nature Communications. doi.org/10.1038/s41467-024-47925-x
Disclaimer: The views expressed here are those of the author expressed in their private capacity and do not necessarily represent the views of AZoM.com Limited T/A AZoNetwork the owner and operator of this website. This disclaimer forms part of the Terms and conditions of use of this website.