Due to their one-dimensional structure and adjustable band gap, graphene nanoribbons (GNRs) have unique electronic and magnetic properties, making them promising for applications in semiconductors, solar cells, transistors, and biomedical devices.
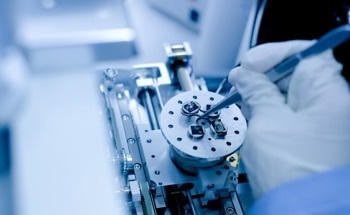
Image Credit: Anucha Cheechang/Shutterstock.com
Introduction to the Formation of Graphene Nanoribbons
GNRs can be synthesized through top-down or bottom-up methods, each providing precise control over their structure and enabling their use in advanced nanoelectronics and spintronics.
Fabrication Techniques
GNRs are created through a series of fabrication techniques designed to achieve specific structural and electronic properties. Lithography, for instance, etches narrow strips from graphene, creating specific armchair or zigzag edges based on a predetermined pattern. This involves manipulating base materials like graphene sheets or carbon nanotubes to tailor desired properties.
Techniques such as unzipping carbon nanotubes lengthwise or utilizing chemical vapor deposition (CVD) to form GNRs with controlled width and edge geometry are well-established techniques. By selecting precursors and controlling parameters, the goal is to achieve desired widths, edge configurations, and properties for various applications.1
These parameters determine the resulting structure and performance of the GNRs, influencing their electronic behavior, mechanical strength, and other critical properties.
Synthesis Methods
Bottom-Up Synthesis
The bottom-up approach constructs graphene from smaller components, typically organic or gaseous precursors. Techniques include CVD, epitaxial growth, pyrolysis, and template-based synthesis.
CVD breaks down hydrocarbon gasses on metal catalysts to form graphene. Epitaxial growth heats silicon carbide to form graphene layers, while pyrolysis decomposes organic materials to create graphene. This method yields high-quality graphene with fewer defects and offers control over structure. However, it requires specialized equipment and is more expensive due to the need for precise control during synthesis.2,3
Top-Down Fabrication
The top-down approach focuses on synthesizing graphene and graphene nanoribbons by breaking down larger materials. It starts with graphite or carbon nanotubes and uses various methods, such as mechanical exfoliation, chemical exfoliation, oxidative exfoliation, and unzipping carbon nanotubes.
Mechanical exfoliation uses tape or an atomic force microscope to remove layers of graphite and produce high-quality graphene flakes. Chemical exfoliation uses oxidizing agents to separate graphite into graphene oxide, which can then be converted into graphene.
Unzipping CNT involves cutting carbon nanotubes to create graphene sheets. These approaches offer versatility but may introduce defects or impurities due to the aggressive nature of some techniques.2,3
Chemical Vapor Deposition
CVD is utilized to create GNRs by depositing carbon-based materials on a substrate and heating it. Catalysts like iron (Fe) can influence the growth pattern and structure of GNRs in CVD processes.
Researchers can adjust growth conditions to produce GNRs with specific widths, orientations, and edge geometries, making CVD crucial for advanced applications.4,5
Molecular Techniques and Edge Engineering
Molecular Self-Assembly
Molecular self-assembly is a critical technique for manufacturing GNRs with precise edge structures. The process combines top-down and bottom-up approaches to fabricate nanoribbons suitable for advanced nanoelectronics, spintronics, and quantum computing applications.
This process involves depositing specifically designed organic molecules, which serve as precursors, onto substrates such as gold or hexagonal boron nitride (h-BN). The molecules are then heated to trigger chemical reactions that allow them to autonomously organize into desired patterns.
The careful design of these precursor molecules is key to controlling the reaction sequence and the resulting structure of the GNRs, enabling the production of tailored, complex nanostructures for various technological applications.1,2,6
Edge Engineering
Edge engineering and control are crucial in materials science. They focus on enhancing material performance by manipulating edges. This is especially significant in 2D materials like graphene, where edge properties affect electronic, optical, and mechanical behavior. Techniques like chemical functionalization and defect engineering are used to modify edge characteristics.
Precise control over edge geometry in graphene can create specific electronic properties for nanoelectronics. Edge engineering can impact mechanical strength and chemical reactivity, providing opportunities to customize material properties.
Scientists aim to develop advanced materials with tailored characteristics through mastering edge engineering. This strategic manipulation of material edges could lead to breakthroughs in fields like flexible electronics and quantum computing.7,8
Post-Synthesis Processing
Post-synthesis processing of GNRs employs a variety of techniques to optimize their structure and functionality.
Annealing methods enhance crystallinity and reduce defects, thereby improving electronic conductivity. Molecular beam epitaxy enables precise growth and functionalization, while polymerization on solid surfaces creates custom GNR structures. Metal nanoparticle-catalyzed cutting provides precise edge control, influencing both electronic and magnetic properties.
These post-synthesis processes are crucial in tailoring GNRs for different applications, from semiconductors and sensors to transistors and biomedical devices. By combining these techniques, researchers can achieve the desired properties in GNRs and extend their use beyond traditional fields.9,10
Characterization Techniques
Characterization techniques are vital for understanding and optimizing GNRs for various applications.
Raman spectroscopy analyzes chemical and electronic structures, helping to identify the edge type of GNRs. Polarized Raman spectroscopy evaluates GNR alignment and quality. Scanning probe methods, such as atomic force microscopy (AFM) and scanning tunneling microscopy (STM), offer detailed atomic-level perspectives of GNR structure and properties. X-Ray photoelectron spectroscopy (XPS) distinguishes different edge types, while electron microscopy provides high-resolution images of GNR morphology.
These comprehensive techniques are essential for the in-depth analysis of GNRs, which are crucial to advancing nanodevices and quantum information systems.11
Applications and Future Directions
GNRs are utilized in a variety of applications due to their unique characteristics.
GNRs are employed in supercapacitors and Li-ion batteries for energy storage, providing high capacitance and improved electron accumulation. They also improve charge transfer rates in supercapacitors and conductive polymer composites used as electrode materials.
Due to their precise structure and electronic behavior, GNRs find applications in semiconductors, transistors, solar cells, and sensors. They are also explored in bio-sensing devices, catalysts, composites, and separation membranes, demonstrating their potential across multiple areas.
The ongoing research and accurate synthesis of GNRs suggest a promising future for them in advanced electronics and quantum computing.10
More from AZoNano: 2D Materials for Environmental Remediation
References and Further Reading
[1]
|
Amogh, K., et al. (2023) On-Surface Synthesis of Edge-Extended Zigzag Graphene Nanoribbons. Advanced Materials. doi.org/10.1002/adma.202306311
|
[2]
|
Shuo, L., et al. (2024) Graphene nanoribbons: current status, challenges and opportunities. Quantum Front 3. doi.org/10.1007/s44214-024-00050-8
|
[3]
|
Aafreen, PV., et al. (2024) Recent Advances in the Synthesis of Graphene and Its Derivative Materials. Graphene - Chemistry and Applications. doi.org/10.5772/intechopen.114280
|
[4]
|
Lou, S., et al. (2023) Tunable growth of one-dimensional graphitic materials: graphene nanoribbons, carbon nanotubes, and nanoribbon/nanotube junction. Scientific Reports. doi.org/10.1038/s41598-023-31573-0
|
[5]
|
Way, AJ., et al. (2022) Graphene nanoribbons initiated from molecularly derived seeds. Nature Communications. doi.org/10.1038/s41467-022-30563-6
|
[6]
|
Tian, C., et al. (2023) Graphene nanoribbons: Current status and challenges as quasi-one-dimensional nanomaterials. Reviews in Physics. doi.org/10.1016/j.revip.2023.100082
|
[7]
|
Yue, L., et al. (2022) Recent progress in the edge reconstruction of two-dimensional materials. Journal of Physics D. doi.org/10.1088/1361-6463/ac855f
|
[8]
|
Takahashi, S., et al. (2023) Effects of Edge Functionalization of Nanographenes with Small Aromatic Systems. ChemPhysChem. doi.org/ 10.1002/cphc.202300066
|
[9]
|
Gu, Y. et al. (2023) Graphene Nanoribbons as Ladder Polymers – Synthetic Challenges and Components of Future Electronics. Ladder Polymers: Synthesis, Properties, Applications, and Perspectives. doi.org/10.1002/9783527833306.ch3
|
[10]
|
Ohtomo, M., et al. (2022) On-surface synthesis of hydroxy-functionalized graphene nanoribbons through deprotection of methylenedioxy groups. Nanoscale advances. doi.org/10.1039/D2NA00031H
|
[11]
|
Mbayachi, VB., et al. (2021) Graphene synthesis, characterization and its applications: A review. Results in Chemistry. doi.org/10.1016/j.rechem.2021.100163
|
Disclaimer: The views expressed here are those of the author expressed in their private capacity and do not necessarily represent the views of AZoM.com Limited T/A AZoNetwork the owner and operator of this website. This disclaimer forms part of the Terms and conditions of use of this website.